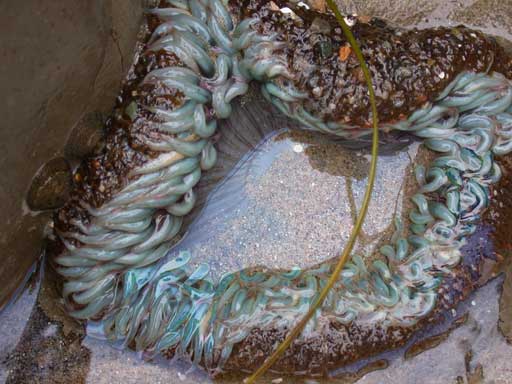
|
BIOL 5130
Evolution
Phil
Ganter
301 Harned Hall
963-5782 |
Anthopleura at low tide |
Adaptation and
Units
of Selection
Lecture
05
Email me
Assignments Page
Back
to:
Lecture Outline
Natural
Selection explains Adaptation
The
Units of Selection
Natural
Selection explains Adaptation
- Ridley argues that directed
change has no evidentiary basis but that evidence does exist for natural selection
as the cause of adaptation
- orthogenesis
is ruled out
- no other mechanistic
explanation
- Not all evolution is
driven by natural selection so not all evolution need result in adaptation
- Lewontin and Gould point
out that a common mistake is to view all features of an organism as adaptations
- drift, hitch-hiking,
developmental constraints, chance, complex fitness landscapes are all
possible reasons for a feature not being optimized
Is
adaptation gradual?
- Rates of
molecular evolution vary over time and within a single gene
- Problem
here is that gradual is not an exact term
- Rate
variation can come as new functions are added or as new interactions between
existing structures are found
- Fisher's
model of an adaptive landscape with one hump leads to the conclusion that
large change is more likely to be maladaptive than a small one
- depends
on simple, unchanging landscape
- Gradual
evolution must overcome the criticism of no value for intermediate stages
- example
of eye has had most attention
- Preadaptation
is also possible
- a structure
serving one function is co-opted for a second function (for which it is
said to be pre-adapted)
- wings
and feathers (especially feathers) are supposed to be pre-adaptations
for flight
- exon
shuffling to make new enzymes
How
good is adaptation?
- Often, adaptation and perfect are in the same
sentence but is that realistic?
- The environment changes but the evolutionary
response can be delayed
- produces a
time-lag in evolution
- will be larger where variation is lacking
in population and mutation is the rate-limiting step
- time lags can lead to extinction
Genetic
Constraints on fitness
- Heterosis leads to continual production of
less fit homozygotes
- Developmental Constraints
- Pleiotropy
- when a gene has effects on more than one phenotypic character
- if the most fit version of the gene for
one character is much less fit for the other, it may not be favored.
- Developmental
Asymmetry
- a measure of developmental disruption
- high for new mutations advantageous
at one locus but somewhat less fit at secondary loci
- if new mutation becomes common, DA
often drops as alleles at other loci that affect the same secondary
traits are selected to reduce the disruption and produce the best
overall phenotype
- secondary alleles are often called
Modifier Genes and the process of returning to the best developmental
pathway is called Canalization
- Canalizing
Selection
is selection for those modifier alleles which are best coordinated
with the advatageous allele at the primary locus of interest
- Morphospace
- the region of possible morphologies
- realized morphospace is the region
of morphospace represented by actual organisms
- the remaining morphospace is unoccupied
- may be due to selective constraint
- may be due to other constraint
(genetic, developmental, mutational, etc.)
- Allometry
- correlated rates of change between different
characters
- by altering these relationships, you can
produce great phenotypic change with very little genetic change
- look at Homo versus Pan, and consider the
small genetic distances involved
- selection of stalk-eyed flies shows very
rapid changes to phenotype but little genetic change
Historical
Constraints on Fitness
- This is linked to developmental constraint
- when change takes place, intermediate forms may
alter the character in a way that is less fit in the current phenotype than
would be if the phenotype had been optimized for current function
- if there are several different, but equally
fit phenotypes possible, then different populations may differ only because
of historical accident and not because the selective topology leads to different
peaks in different places as is often assumed
Functional
Constraints on Fitness
- some changes may be linked to other aspects of
the organism's function (e.g. larger bones are stronger and will resist breakage
but also weigh much more and will cost more resource to carry around and maintain)
- This is an example of a
Phenotypic Trade-Off and trade-offs may constrain evolution
of a trait
Adaptation
as Design and as Fitness
- Adaptations are often discussed as advances in
design
- position of eyes in raptors versus waterfowl
- raptors eyes face forward and allow two eyes
to focus on all objects of interest (often called binocular vision)
- good for spatial perception and raptors can snatch
a prey item while at full speed flight
- ducks do not focus both eyes on food items but
they do have much wider fields of vision
- they are more often prey than raptors and the
wider visual field gives them advantages over raptors in detecting approaching
predators
- This is an example of adaptation as design as
the advantage is a direct result of changes in design
- Fitness is still the coin but it would be very
hard to test the fitness differences as all raptors have binocular vision
throughout their visual field and waterfowl do not, so how to compare as many
other differences also exist between the two groups
- Some of the "Adaptationist Agenda"
from the Spandrels article results from assumption that designs are always
perfect and that differences must therefore be fitter and so the design is
better, which is circular
The
Units of Selection
What is favored by selection?
- The gene?
- The organism?
- The population?
- The species?
All have been argued to be the beneficiaries of
natural selection but can they all be?
- What happen when a benefit to one level is a
cost at another (when selection at different levels is opposed)?
- Answering this question has provided evolution
with one of its most long-standing controversies
Genic
Selection
- selection that increases the number of copies
of a gene in succeeding generations, no matter what the effect on other levels
- Example of genic selection is the phenomenon
of meiotic drive genes (segregation
distorter genes)
- t-allele of mice (simplified)
- t-allele (actually more than one closely
linked loci), when homozygous, produces sterile males
- when heterozygous in male mice it produces
a short-tailed mouse that have the t-allele in 90% of their sperm (not
the expected 50% so this is the meiotic drive or segregation distortion)
- genic level selection favors the distorter gene
but organismic selection favors the wild type allele (haplotype) and it is
also not favored at the group level as, in small populations, if all of the
males become t-allele homozygous there is reproductive failure (the groups
have higher than average probability of extinction).
- The actual frequency of the t-allele is a
balance between the three levels.
- meiotic drive alleles have been discovered in
lots of insects, some fungi, and some plants so it is a widely spread phenomenon
- the logic behind a 1 to 1 sex ratio being the
stable strategy involves selection for genes that over-produce the rare sex
Cellular
Selection
- This is the outcome of different cell lines reproducing
independently
- many animals have a distinction between the germ
line and the somatic cells
- only mutations to the germ line cells have
any chance of being inherited in this system
- however, most other organisms have no such distinction
and a mitotic mutation may lead to a part of an individual that gives rise
to some reproductive tissue, which will carry the mutation, although other
reproductive tissues on the same individual will not
- thus, cell lines can be selected independently
of the individuals within which they reside
Organismic
(Individual) Selection is what we have been studying up until
now
- This can get complicated when you consider what
an individual organism is
- What is an organism when parts can become separated
and reproduce independently (plants connected by rhizomes can be separated
and each part is a new plant)
- some colonial animals are similar (cnidarians
are a good example)
Group
Selection
- When group and individual and genic selection
all promote the same alleles, joy reigns in mudville
- When group and individual selection are opposed,
many believe group selection will always yield to individual selection
- the trait which harms the individual but
helps the group is the Altruistic Trait
Altruism
can lead groups with individuals that have it to found new groups faster than
groups lacking individuals with the trait
- This is group selection
- however, those individuals in the group containing
individuals with the altruistic trait but which lack the trait themselves
will be favored within the group
- they do not pay the cost of the altruism but
they reap the benefit
- so altruistic groups are vulnerable to invasion
(by immigrant individuals or through mutation to the genes for altruism)
and are, eventually, taken over by the slackers
- Group selection among family groups
Kin
Selection
- helping a relative costs an individual some
or all of its reproductive output
- the relative boosts its reproductive output
through the help (the benefit)
- because the individual is helping a relative,
it can be sure that some of the genes in the relative will be identical
to its own genes
- when the relative reproduces, it is reproducing
the helper individual's genes, which means the helper gets benefits
- how sure it can be of this depends on how close
a relative is being helped, so there must be a discounting of the benefit
for more distant relatives
A different model of Group Selection (from Sober and Sloan Wilson, Unto
Others, 1998)
- There is a locus which determines altruism (A) or selfishness (S)
- the gene frequency of A is p, of S is 1 - p
- in a group, there are n individuals, np of which are altruists and n(1
- p) which are selfish
- Without altruism in a group, all individuals, no matter what genotype, average
x offspring
- altruists cost themselves c offspring but help every other member of the
group to have b additional offspring
- the fitness of each genotype is
WA = x
- c + (b(np -1) / (n - 1))
WS = x
+ (bnp / (n - 1))
- Altruists lose the cost of altruism but benefit from the presence
of other altruists, each of which provides a benefit, b, so the total for
the altruist is b(np - 1) total benefit spread over n - 1 individuals in
the population
- Selfish individuals have no costs and greater benefits, as
they benefit from all altruists (each altruist can not benefit itself, or
it wouldn't be an altruist)
- We can use a numerical example to show that this model can
select for altruists when there are more than one populations in the metapopulation
x = 10 |
|
|
|
c = 1 |
|
|
|
b = 5 |
|
|
|
|
Group 1 |
Group 2 |
Total |
Population Size |
100 |
100 |
200 |
p = |
0.2 |
0.8 |
0.5 |
1 - p = |
0.8 |
0.2 |
0.5 |
Altruist fitness |
9.96 |
12.99 |
|
Selfish fitness |
11.01 |
14.04 |
|
|
|
|
|
Next Generation |
|
|
|
n' = |
1080 |
1320 |
2400 |
p' = |
0.184 |
0.787 |
0.516 |
- From the above, you can see that:
- the fitness of altruists is less than that of the selfish individuals
in each subpopulation, as expected
- the frequency of the altruist decreases in each subpopulation,
as expected
- the frequency of altruists in the total population INCREASES,
which is definitely not expected
- the global increase is due to the relative grow rates of the
subpopulations
- the "altruist" population grows much faster, due to the effort
of the altruists
- where selfish individuals predominate, the populations grow
less
- the overall effect is to boost the overall frequency of altruists
(globally only)
- Sober and Wilson are propose that this effect (known as Simpson's
Paradox) may be behind the evolution of altruistic behavior
Last updated February
27, 2008