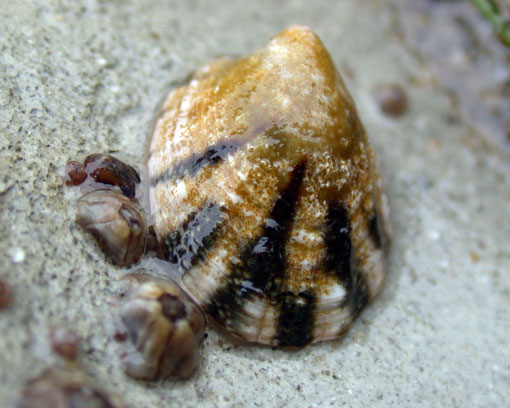
|
BIOL 4140
Contemporary Problems
in Environmental Science
Phil Ganter
302 Harned Hall
963-5782 |
A limpet and acorn
barnacles |
Energy I: Energy Basics and Non-Renewable
Energy Sources
Chapter 7
Email
me
Unit
Organization:
Reading:
Textbook: Chapters 7
and 8
Ancillary Reading:
Energy and Its Measurement
Energy and
Measuring Energy
Energy
has the worst definition of any "thing" I know of. No one
I know seriously denies it's existence but no one has ever been able to tell
me what it is. So, you won't be getting a better definition from me
unless I am struck by a thunderbolt (electromagnetic energy, I believe).
- "the
ability to do work"
-
What
is work?
- Work
is done when you force something to move some distance, like driving your
car down the block (the engine
does the work, you just add to its work)
or forcing
ions across the cell membrane of a neuron (which is why thinking is work,
real
work)
- We
won't go into a definition of distance here but a definition of force will
be needed if we are to understand work
- Force
depends on the mass of the
object being moved and the acceleration to
which it is exposed.
- We
measure mass in grams (or a multiple)
and acceleration
in meters per second per second.
- If
we accelerate 1 kilogram of mass 1 meter
per second per second, we are applying
1 newton of force. Back
to work:
W
= Fd, or work = force times distance
- So,
if we apply 1 newton of force and move something a meter, we have done
1 joule of work
- Energy is measured in the same units as work,
so one joule of energy expended to do the work described above but energy
is not the same as work
- Energy,
not work, can be stored as potential energy
- Energy
is found in many useful forms: photons, kinetic energy, and various
kinds of potential energy (a chemical bond, an electrochemical gradient)
- Energy efficiency is simply the proportion of work done divided by the
energy expended (or by the energy put into the system)
- Power is related to energy. Power is work per unit time and is measured
in watts (1 W = 1 J per second), so every kilowatt (kW, 1000 watts) uses
up
There
are several systems to measure energy and you might see any of them used. In
addition, you should get some feel for the units used for large-scale descriptions
like US or global energy consumption.
- Joule (J) or erg (for global purposes, the petajoule (PJ) = 1015 J)
- these are in the metric system
- Calorie (cal,
= 4.184 joules) or kilocalorie (kcal) - the calorie listed
on food packages is actually a kilocalorie
- British
Thermal Unit (BTU =
1055 joules or 3.968 kcal) or Quad (quadrillion BTUs,
1015 BTUs
- could also be called a petaBTU but that would be mixing metric prefixes
with non-metric measurements)
- When
reading graphs or tables about worldwide energy consumption, PJs or
Quads are used (1 Quad is about 1000 PJs)
- Metric
Tons of Oil Equivalent (toe) - a metric ton is
1000 kilograms (about 2,200 lbs) and the energy in one metric ton of oil
is about 41.9 x 109 joules
and a million toes (Mtoe) is about 41.9 PJ or 0.04 Quad
- more
on oil - a barrel (bbl)
of oil is 42 gallons or 159 liters and a liter is about a kilogram
[a bit less, as oil is not as dense as water], so one
metric ton
of
oil is about 6.3 barrels of oil
- US
consumption of energy in 2010 was about 98 quads, which translates into
24.5 billion barrels of oil
- However,
only about 26 of the 98 quads came from oil, so actual oil consumption
was about 6.4 billion barrels, or about 19 billion barrels per
day (remember,
this
is just in the US), most of which goes into transportation)
Global Energy Consumption
- Total
energy consumption is subject to the general rule for global impact:
impact
is equal to consumption times population:
I
= C x P
- So
increases in consumption can be due to both increasing population (we know
this is still occurring) or to increases in per capita consumption of
energy
- As
the economies of Brazil, Indonesia, India and China grow, the per capita
energy consumption of their citizens grows, and this fuels global per capita
growth
Update
of the Book - the global recession caused a reversal of the usual increase
in energy consumption, but the recovery as restored the historical trend of
ever-increasing energy consumption
Electricity
Generated
by many energy sources, it is the real energy “currency” and, if
electric vehicles become the norm, its status will be further enhanced
- Fossil
fuels (coal, petroleum, gas)
- Nuclear
fuel
- Waste
- Renewable
Fuels (Geothermal, wind, solar, Hydropower)
A
large portion of the energy sector is devoted to supplying consumers with electricity
to run electrical appliances, lighting, heating, and so forth
- Most
commercial power plants are described by the type of primary energy used to
operate the turbine-driven generators.
Industry
is taking a bigger bite of the global total
- Now
24%, up from 20% in 2008
- Industry
is becoming more efficient globally as technology goes global
- The
energy needed to produce 1 unit of “added value” by industry
is dropping worldwide
- 1.
Industrial energy consumption is getting more efficient worldwide
- 2. Efficiencies
are becoming more uniform as modern methods spread
Electricity
Generation
Michael
Faraday is credited with discovering that a charged particle will be pushed
along by a moving
magnetic field (and vice versa)
- This
principle of electromagnetic induction is the basis for both electric generators
and motors
- The
generator in your car is a small version of the generators in power plants
Magnetism
- A
magnetic field is generated by the motion of a particle with an electric charge
(for instance, an electron)
- No
motion, no field but electrons are always in motion
- Some
atoms have electron arrangements that give the overall atom a strong magnetic
field
- Fe,
Co, and Ni are the common ones at room temperature
- Only
unpaired electrons contribute to the atom's magnetism
- pairs
are always moving in opposing directions, thus canceling each other out
- Magnetism
(a coherent magnetic field around an object) can be induced in some materials
with moderately magnetic atoms by applying a current so that the magnetic moments
of the component atoms align
- The
effect of the individual atomic “magnets” adds
up
- Some
atoms have electron configurations that give the overall atom a magnetic field
and a crystal structure that encourages them to form magnetic “domains” – clusters
of aligned atoms (very small but with about a quad of atoms in each domain)
- If
the domains in a bar of iron are in alignment, then the entire bar has a coherent
magnetic field
- One
way to get them to align is to put them into the field of a pre-existing
magnet!
Electromagnetic induction
- caused by moving a magnetic field
past a copper wire (or some other conducting material)
- A
lot of electrons moving in the same direction down a wire is a current
- the
energy in this movement can be used to do lots of different kinds of work:
heating, computing, etc.
- The
magnetic field will cause the “free” electrons
in the
conductor to move according to the “right hand rule”.
- So,
how do we get the magnet to move?
- just
spinning it is movement enough
- We
need to turn a shaft fixed to the magnet
- This
turning is accomplished by a turbine
- A
turbine is a mechanism composed of blades attached to a shaft that, when fluid
(steam, often) flows by them,
are pushed so that they turn the shaft.
- If
you run this in reverse, you have a compressor or a pump!
- A
windmill is a kind of turbine
Electrification
Two sources:
- Grid Electricity - electricity generated from a
central power plant and distributed to many users through wires
- The wires form a grid that interconnects
power plants
- The Grid
- Grew as local power companies extended their
serivce areas and began to interconnect power lines for improved stability
- Is
managed by agencies (called "Balancing Authorities") set up by groups
of power companies
- Balancing Authorities constantly monitor
demand locally and call up local and distant generation plants to satisfy
local demand
- The normal priority is price-based (cheapest
used first) but during peaks, other criteria are used (all suppliers
get paid at the rate that the most expensive supplier receives)
- In the future, environmental costs may
become part of the prioritization criteria (call up polluters last)
- The Grid may be the most complex machine
on Earth
- Individual generators or solar cells that supply
electricity directly to a single user.
Supplying the People with Electricity
- Electrification of the US
- By the 1870's, electrical generators were for sale
in Europe and the US
- In 1879 in the US (Edison) and 1880 in the UK (Swan),
incandescent light bulbs were patented
- So, now there was a means to generate electricity
on a large scale 24 hours a day and a practical use for that electricity
in every home, office, and factory: lighting
- In America, the focus was on direct current (DC)
- where the current always runs from the source (generator or battery) to
the
user
- In Europe, alternating current AC was favored -
current that would reverse directions constantly
- Serious electrification of cities started in the
US in 1905 and, by 1930, 70% of urban households were electrified
- In 1930, only 10% of rural America was electrified
- Low population density lead to slow penetration
of the grid into rural areas because privately- owned electric companies
thought it too costly
- 1935 - REA (Rural Electrification Administration)
was established by the US government
- REA established rural non-profit cooperatives as
the means of electrification
- Roosevelt initiative - opposed as unfair competition
with private enterprise
- 1940 - rural electrification at 33%
- Electrification of the World (2009 data, percent
of total population)
Region
|
Urban |
Rural |
Overall |
North Africa |
100 |
94 |
99 |
Sub-Saharan Africa |
60 |
14 |
31 |
China and East Asia |
96 |
86 |
91 |
South Asia |
69 |
60 |
68 |
Latin America |
98 |
74 |
93 |
Middle East |
98 |
72 |
89 |
Other Developing Countries |
91 |
63 |
75 |
World (both Developed and Developing) |
94 |
68 |
81 |
- Proportion of those served by the grid is always
less in rural areas
- Low population density means every user must get
more wire which increases both cost to set up wire and to maintain it
- Proportion of those with access to the grid varies
in different regions
- In sub-Saharan Africa, the rate of electrification
has been lower than the growth rate, so that the proportion served by the
grid has been declining
A large portion of the energy sector is
devoted to supplying consumers with electricity to run electrical appliances,
lighting, heating, and so forth.
Power Plants
- Most commercial power plants are described
by the type of primary energy used to operate the turbine-driven generators.
- Fossil fuel plants boil water and use the steam
pressure to drive the turbines
- These can be coal, oil, or natural gas
- Natural gas can sometimes be liquefied
after being pumped from wells
- Liquids are safer to transport and store
- Nuclear
Power Plants are similar to Fossil Fuel Power Plants in that they heat a
fluid and use the heated fluid to drive a turbine
- Waste-fueled Electric Power Plants burn garbage
to generate electricity
- Must be very careful to remove toxic
materials from their emissions as it is difficult to predict just
what will be in the waste burned
- Hydroelectric Power Plants use the pressure
of water to drive the turbines
- Solar Power Plants directly convert photons
of light into energy
- Geothermal Power Plants heat the water with
heat from below ground
- Wind Farms concentrate wind turbines in
a single location and combine their individual outputs
- Most utilities that generate electricity must have a larger generating capacity
than is actually needed
much of the time.
- The surplus capacity provides a reserve to meet fluctuation in peak demand
or to cover repair and maintenance downtime for power plants or portions of
the electrical grid.
- Brownouts and blackouts can occur that disrupt society if supply of energy
exceeds demand.
- Blackouts are total loss of power for a
group of users
- Brownouts are drops in voltage for a group
of users (the lights are less bright, the heater will not get as hot,
your toast will take forever to get toasty)
- brownouts can
be as bad as a blackout for industry and for the operation of critical
infrastructure
(water pumps, medical equipment, etc.)
- Annual electric generation has climbed steadily since 1980 and the proportion
from fossil fuel burning has remained steady
Hard and Soft Technologies
- Hard technologies: large-scale plants, which are complex, expensive, and centralized
- Large power plants fueled by fossil fuels, water, or atomic energy
- Some “renewable” hard technologies:
Large scale solar plants, windmill farms
- Soft
technologies: small-scale plants and applications, which are local, and usually
more environmentally friendly than hard-energy technologies.
- active and passive
solar on a single building, individual windmills, solar panels for individual
lights, etc.
- Most electricity is generated by large “hard tech” plants
Fossil Fuels
The
fossil fuels (coal, oil, natural gas) formed over geological time from the
remains of plants and animals buried under layers of sedimentary rock.
- People
are consuming fossil fuels millions of times faster than they are
forming, so we consider these to be Non-Renewable.
Have
fossil fuel supplies increased or decreased over time?
- Known
reserves may increase if we discover more deposits
- The
total supply may decrease even as more deposits are found if we use the resource
faster than we add to the reserves
- How
long the reserve lasts is related to both the total amount of resource that
can be recovered and the rate of rate of energy use.
- If
you want it to last a long time, assume "at current rates of use" and
you will predict a long period before the resource is depleted
- If
you assume a constant, positive growth rate, then the time-to-depletion will
be much shorter (even for modest growth rates)
Oil
Regional
oil production and consumption
Region |
Production (%)
|
Consumption (%)
|
Middle East
|
30
|
8
|
Russia |
15
|
5
|
Africa |
13
|
4
|
US |
10
|
23
|
Asia and Oceania |
10
|
29
|
Central and South America |
9
|
7
|
Canada and Mexico |
8
|
5
|
Europe |
6
|
19
|
Just how much of it is there?
- Must
be a finite resource!
Four
components of this question:
- What
we have used up to now?
- What
we know about (Proven Reserves)?
- What
we have not yet discovered?
- What
we will never discover or never use?
Hubbert’s
Curve - M. King Hubbert devised a way to use
the first two to predict the last two
- Uses
historical production and proven reserves to predict future production
- Bell
shaped curve
- Curve
first rises due to
- new
discoveries
- new
infrastructure
- Curve
peaks and declines due to
- Reduced
discovery rate
- Resource
depletion
- Implications
of Hubbert’s Curve
- We
must expect to live with declining production of some resources
- Economic
systems based on continuous growth (exponential growth) will fail in a world
of declining resource
- What
is the interaction between price of oil and the implications Hubbert’s
Curve
- Price
may increase the incentive for
- Discovery
- Efficiency
- Conservation
- But
it will not increase the amount of the resource
- Oil
Production and Price
- Oil
production has been much more steady than price
- Market
is not supply and demand but anticipated supply and demand
- Oil
Demand and Discovery
- In
economics, supply is usually tied to demand, such that greater
demand will result in greater supply
- Oil
demand has continued to increase but discovery of new oil has declined, not
increased.
- The
gap is ever growing as we now consume more than we discover each
year.
- What
is the interactions between technology and the implications of Hubbert
Curve?
- Technology
may increase:
- Rate
of discovery
- %
of resource that is useable
- Efficiency
of resource use
- But
these will not increase the amount of resource
- Technology
may provide a substitute for the resource (Nuclear Fuel or Wind for Coal and
Oil)
- This
is how technology can alter the outcome
- However,
the substitute may also be governed by Hubbert’s curve
- One effect of Hubbert's curve is
that, as easily extractable oil (lighter, more runny) becomes
less available, petroleum once too expensive to extract and
refine becomes cost-effective
- There has been a marked rise in
extraction of thick, bituminous petroleum since we
passed the peak in the Hubbert Curve for world oil production
- Hubbert’s
reasoning can be applied to any finite resource and there
are peak curves for:
- Natural
Gas
- Coal
- Phosphate
(fertilizer component)
- Nuclear
fuel
- Transition
metals (Cu, Ni) and Precious metals (Au, Ag)
- A
modified form of the curve can be applied to renewable resources IF:
- the
resource is being overexploited in that it is being harvested much faster than
it is being renewed
- Fresh
Water and Oceanic Fish are two examples of over-exploited resources
Coal
Coal
is much more abundant than oil and more evenly distributed throughout the world.
- Mostly
used to produce electricity (some for steel production and some for heating)
- There are four kinds of coal that make up a
series from most plant-like to most altered by pressure, heat and time
- quality is related to how much energy one can
get per kilogram burned and how much impurity is present
- Peat - decayed, pressed plant remains (not usually used for electricity
production)
- Lignite - low-quality coal, soft and often brown
- Bituminous Coal - mid-level quality, soft, contains a tar-like material
called bitumen
- Anthracite - highest quality but almost all has been mined (only PA
mines it in the US), black, hard and shiny
- Coals origins
- Coal comes from almost all geological periods (even some Pre-cambrian,
which must be from algae since plants had not yet evolved)
- Carboniferous Coal - Eastern US
- Permian coal - Russia and Australia (which also has coal from the mesozoic)
- Major coal producers are China (48%), US (15%), India (6%), Australia (6%),
Indonesia (5%) and Russia (5%)
- Major coal reserves are in US (22%), Russia (14%), China (13%), Australia
(9%), India (7%) and Germany (5%)
- In the US, there are five coal regions (Eastern, Gulf, Interior [IN,
IA, IL, MO], Rocky Mtn, and North Plains
The
world’s known coal supply could last about two hundred years at
current consumption rates
- much less time if it is used as a replacement when the oil
resource is exhausted.
- time-to-depletion
is also shortened by increased demand for electricity that is met by increased
production
Why
the internet is a dangerous place for the unaware
- "It
has been estimated that there are over 847 billion tonnes of proven coal reserves
worldwide. This
means that there is enough coal to last us around 118 years at current
rates of production. In
contrast, proven oil and gas reserves are equivalent to around 46 and 59 years
at current production levels.”
- 2011
World Coal Association http://www.worldcoal.org/coal/where-is-coal-found/
- The
webpage defines “proven” but never indicates how the time
until depletion is calculated.
- Are
they allowing the rate of consumption to grow?
- Would
you accept their figure of 118 years if they do not assume increasing demand?
Natural
Gas
Mixture
of volatile hydrocarbon molecules
- Methane
most common, ethane second most common
- Source
for propane, butane, pentane, hydrogen sulfide (can be processed into sulfur),
and helium
Formed
naturally in two ways:
- Thermogenic gas
(in deep rock where organics are converted into simple, reduced hydrocarbons
under great heat and pressure)
- Thermogenic
gas deposited underground
- Trapped
in porous rock under impermeable rock layer
- Often
(not always) found with oil or coal deposits
- Biogenic gas
(metabolic product of a prokaryote from anaerobic respiration)
- Biogenic
gas is generated in:
- Wetlands
- Flooded
soils have little O2 so
decomposition goes anaerobic
- Landfills
and Sewage Sludge
- Sludge
is the product of sewage treatment
- Cows
- Gut
symbionts produce methane
Town
Gas:
- Gas
produced from coal (by heating in closed ovens -- not economically feasible
today)
- Cities
would build a gas-plant and pipe the gas into homes for cooking and heating
Natural
gas is obtained by
- Collecting
it from wellheads and piping it to customers
- First
processed to remove contaminants (H2S, mercury, water, CO2, N2 and
others) and separate valuable
minor components
- To
release the gas from rock with very small pores, some wells are first “fracked”
- liquids
(water, mostly) are pumped at very high pressure into the well to
fracture the rock, releasing the gas
- at
least some fracking fluids must be pumped back up to facilitate release of
the gas
- Natural
Gas Plant Liquids (NGPL)
- Higher
molecular weight hydrocarbons are turned into liquid fuels
- Much NGPL is used to dilute the bitumen
and other viscous, high density petroleum that are taking the place
of the easy to extract (and now mostly gone) lighter, liquid crude
oil most of us think of when we think of oil
- Imagine a "gusher", a well spouting oil
- much of the "oil" now pumped is too thick to gush
- NGPL is a source of cheap light-weight hydrocarbon
that can be used to dilute the thicker oil well products so that they
will flow through the pipelines used to transport petroleum to refineries
- The mixture of the tar (properly called
bitumen) and NGPL is called Dilbit (DILuted
BITumen)
Environmental
Hazards associated with Natural Gas
- methane
is a more potent greenhouse gas than CO2 so
any release of gas into the atmosphere contributes to increasing the greenhouse
effect
- natural
gas
combustion produces CO2 the
primary greenhouse gas
- natural
gas contains contaminants that are pollutants both before and after burning
- H2S
and derivatives (mercaptans), siloxanes, mercury, and radon
- H2S
is poisonous, radon is radioactive
- Burning
CH4 oxidized H2S into SO2 and SO3, which combine with H2O in the atmosphere
and form Sulfuric and Sulfurous Acid and so acidify rain
and lakes
- fracking
fluids contain pollutants and must be treated before release
Methane
Hydrates / Clathrates
- Formed
from water and methane at high pressure and low temperature
- Methane
is trapped inside of the ice crystals (not always normal ice crystal
structure)
- Found
in Permafrost and in Marine sediments at ocean bottom
- Known
Hydrate Deposits are off of coasts throughout the world
- Huge
amount of methane (probably biogenic) trapped in these deposits (may
be more than is in rock deposits)
- Some estimates are that 2/3 of all carbon
in oil, coal, and natural gas is in these methane clathrate deposits!
- Some
see it as a potential resource
- Some
see it as a potential hazard because, if released, methane is a more potent
greenhouse gas than CO2
Concerns about Fossil Fuels
- Energy
consumption raises two basic concerns:
- How
will we power our future as we deplete current energy sources?
- How
can we minimize energy-related environmental degradation?
- Historically,
energy-related problems have been addressed by using more energy.
- A
more productive approach to these problems may lie in reducing demand by
increasing efficiency and/or by conservation.
- Efficiency
of energy extraction for ways of generating electricity
- Hydro
reaches over 90% efficiency
- Of
the fossil fuels, natural gas is best (55%),
- Wind
similar to fossil fuels
- Solar
now near 20%
the ANWR
controversy
Arctic
National Wildlife Reserve
- 19
million acres
- Largest
wilderness area in the US
- Important
for preservation of several species, including a subspecies of Caribou (Porcupine
River)
- Canada
has large wilderness areas bordering on ANWR
- Drilling
is proposed for the “1002” Area on the coast of the Arctic
Sea
What
would we get from drilling?
- Hard
to estimate at the present but
- 1998
estimates were between 5.7 and 16 billion bbl (4.3 to 11.7
on Federal Land)
- 2010
estimates of oil from nearby coast were far under previous estimates as many
test wells produced natural gas, not oil
- Productivity,
if it were opened to exploration and drilling now, would begin in 2018, peak
(using the mean reserve figure) in 2027.
- Production
at peak would be 0.78 million bbl/day
- In
2010, US DOE says, we produce 5.5 million bbl/day and consume 21.3 million
bbl/day (the gap is filled by imports and other liquid fuels)
- So,
ANWR will never be more than 3.6 % of US need (6.8% for highest reserve estimate)
What
about gas prices?
- Gas
prices are set by an international market, not the US market
- ANWR
production will never be more than 1.2 % of global consumption (high estimate)
and could be less if demand increases faster than predicted
- So,
it would mean, at today’s $3.60
per gal price, a difference of 2.8 cents per gallon, assuming 65% of gas price
is due to oil price
the Future
of energy production
- At
present, the environmental impact of our energy use is straining—and
perhaps has exceeded—the capacity of natural systems to maintain themselves.
- Reducing
the energy-induced environmental destruction will require reducing energy consumption
by increasing efficiency and increasing the use of alternative energy sources
that reduce the environmental impact of energy production.
- The
Federal Government subsidizes energy sector industries
- This
makes price a poor predictor of future contributions from various energy options
- Market
economics may not find the optimal solution to the question of where we should
get our energy as:
- price
in the market now only reflects production and distribution costs, not environmental
costs
- subsidies
distort prices
- Distribution
of subsidies in 2002 to 2008
- Fossil
fuels:
- $72,500,000,000
(72%) -
- $2,300,000,000
(3%)
for environmental cleanup efforts (carbon capture and storage)
- $70,200,000,000
(97%)
for tax breaks and other support
- Renewable
energy
- $12,200,000,000
(28%)
for solar, wind, geothermal, etc. support
- $16,800,000,000
(28%)
for corn ethanol support
- All
in all, government support for sustainable, renewable, non-food crop energy
is only 12% of total subsidies for energy
- Governments
(many national governments, US federal and some US states) are debating
taxing various energy sources for their particular environmental costs
- Oil
spills, land and water contamination by coal mining, carbon dioxide emission,
acid rain, etc.
- Normally,
environmental costs are not paid by the energy consumer alone but by everyone
- Environmental
impact Taxes
reflect the environmental costs and should be spent for remediation
- Environmental
impact taxes are a means of putting burden on the consumer, so that
the consumer will choose among energy sources based on the total cost
of
the energy from each source
- Tennessee
already has this sort of scheme in place at the gas pump but not
for assessing the cost of environmental impact
- Gas
tax is to pay for building and maintaining roads
- Public
roads are a cost to all and our gas tax in meant to shift some of the burden
of paying for them from the general public to those who use Tennessee's public
roads
- Environmental
Impact Taxes use the same logic as the "user-pays" gas tax
- Carbon
tax is the most commonly discussed but it is only a specific example of a general
principle
- Tennessee’s
gasoline tax (From http://www.tdot.state.tn.us/gastax)
- 21.4
cents per gallon (20 gasoline tax + 1.4 special petroleum fee)
- Yields
$668.9 million per year
- How
the tax is divided
- 7.9
cents, or $246.8 million, goes to cities and counties
- Approximately
.7 cent, or $22.1 million, goes to the State General Fund
- Approximately
12.8 cents, or $400.1 million, goes to TDOT
- The
$400.1 million is included in TDOT's total state revenue of $874,300,000 and
is used in three basic ways to accomplish TDOT's mission:
- Basic
operating costs
- Highway
maintenance contracts
- Resurfacing,
bridges, major reconstruction, new construction, consultant contracts, right-of-way
purchases, and to match federal funds
- Currently,
most Gas Taxes in various countries and states are not Environmental
Impact Taxes
Why
We Must Stop Burning Fossil Fuels (from textbook)
- "Whether
or not we are in danger of running out of fossil fuels, we must curtail their
use
- The
use of coal, oil, and natural gas facilitated the development of modern industrialized
society but it has also created environmental havoc on a global scale
- While
the duration of existing and possible reserves is unpredictable, the major
concern is the large-scale environmental degradation that using fossil fuels
creates."
Politics
and Energy
- This
is another "wedge issue" in our political life and, as such, you should be
very skeptical of many websites with material on the subject of energy and
energy policy
- One
small example:
- A
comparison of the change in US per capita and Chinese per capita energy use
- One
site has a graphic of US versus Chinese usage over recent years
- If
one does not look at the axis labels closely, one might think that the average
Chinese
citizen
now consumes more oil than the average American
- But
a graph with both rates are on the same scale shows that, although Chinese
per capita usage has gone up recently, US per capita usage is far, far more
than Chinese per capita usage
- So,
why the scare tactics when the average Chinese citizen sips oil and the average
US citizen gulps it?
- The
size of China's population means that their total demand has exceeded US
demand
- So
China is now competing for resources with the US and has replaced Japan as
the “Eastern Economic Threat”
Environmental
Impact of Coal (an example from our textbook)
- Using
more coal can increase air pollution
- Coal
emits 25% more CO2 than an equal amount of oil
- Coal
emits 80% more CO2 than an equal amount of natural gas
- Burning
coal releases sulfur dioxide and nitrogen dioxide, the major contributors to
acid rain
- Environmental
Impacts of the Coal Fuel Cycle (see diagram in textbook)
Energy
Use Impacts
- The
fossil fuels (oil, coal, and natural gas) are the major source of air pollution,
acid rain, and greenhouse gases.
- Mineral
extraction of fossil fuels and uranium, and dam construction for hydroelectric
power, both create major environmental destruction
- The
conventional fossil fuels, upon which we rely heavily, are nonrenewable and
are being depleted rapidly.
Nuclear Power
Nuclear
Power (from textbook – pre Japanese tsunami disaster at Fukushima)
- Nuclear
power supplies about 6.5% of the world’s commercial energy, but supplies
a much larger percentage of electrical generation in some industrial countries.
Growing
public concerns about safety, and the high cost of nuclear power, are changing
the earlier enthusiasm for nuclear-generated electricity in many countries.
- Since
1978, there have been no new nuclear plants ordered in the U.S. but other
countries like China and India have continued developing their nuclear capacity.
Principles
of Nuclear Power by Fission
Both
the fission and fusion of nuclear power involve rearranging the structure
of atoms.
- Fission
is the splitting of an isotope of a heavy element into daughter products
(smaller atoms) with the release of energy.
- All
commercial nuclear energy generation is by fission.
- Fusion
is the joining of isotopes of a light element into a heavier element with
the generation of energy.
- The
sun generates heat and light by fusion.
- Some
nuclear weapons use fusion reactions but controlled fusion is still in
the development stage and has been for 50 years.
Nuclear
Radiation
- Radioactivity
comes from Radioactive Decay of unstable isotopes
- Light
is radiated energy, so what do we mean when we speak of atomic radiation?
- Unstable
atomic nuclei change to become less unstable or stable (Second Law says they
must)
- There
are three general types of radiation (Alpha [
],Beta
[
],
and Gamma [
]) related
to the three general causes of instability:
- Instability
due to excess of protons resulting in too much repulsion within nucleus
- Alpha
(
) emission is the ejection of a helium nucleus (two protons and two neutrons)
- there are other, rarer, forms of
proton loss
- Instability
due to unstable neutron:proton ratio
- (Beta,
) – electron (
-particle)
emission leaves behind a proton, which changes the element to which the atom
belongs
- (Beta) – positron
emission
- (Beta) – electron
capture when an electron orbiting a nucleus is captured and
fused with a proton to form a neutron
- note:
no radiation produced but a proton is converted into
a neutron so the atom becomes a different isotope of the
element to which it belongs
- there are other, rarer forms of
ratio adjustment
- Instability
due to excess energy in nucleus
- Gamma
(
) emission releases a very
high-energy photon (only cosmic rays have more energetic photons)
- Different
kinds of radiation (emissions) have different effects
- Size,
charge, and weight of particle determine how likely it is to interact with
(hit) another atom
- the
addition of the collision energy (or absorption of the gamma photon energy)
can break bonds between atoms in molecules (like DNA), causing metabolic
damage (this is the danger of radiation)
- Different
types of radiation have different powers of penetration
- Alpha
particles will all hit atoms in a sheet of dense paper,
- beta
particles can penetrate paper but a thick layer of plastic will
stop them
- gamma
photons penetrate most matter an only a thick layer of
dense material will absorb them (lead is very dense)
- Once
ingested, danger reverses!
- Alpha
particles emitted in the stomach will cause havoc in the lining
of the stomach
- beta
particles emitted from material in the stomach will penetrate
deeper
into the body
- gamma
photons emitted from material in the stomach will most probably
pass out of the
body without doing any
damage
- Radiation
exposure
- An
average US citizen receives, during his or her
life, potentially damaging radiation from these sources:
- 50%
of radiation from radon in the environment
- 15%
from medical treatment or diagnosis
- 13%
from untraceable gamma sources
- 12%
from cosmic rays (more damaging than gamma)
- 10%
from ingested radioactive isotopes
- less
than 1 % from all other sources (fallout, commercial products, improperly
disposed of radioactive materials, etc.)
- These
% can change radically for individuals caught near a nuclear disaster
- Radioactive decay happens as a series of emissions
- exact
steps are different for different fissile elements but lets look at 235U92,
the most commonly used isotope in both bombs and reactors
- 235U92
starts not as uranium but as plutonium (235Pu94 ,
[24,300 yr])
- Pu - alpha emission to Uranium (235U92,
[700,000,000 yr])
- alpha decay to Thorium-231 (231Th90,
[25 hours])
- beta decay to Proactinium-231 (231Pa91,
[32,000 yr])
- alpha decay to Actinium-227 (227Ac89,
[21 yr])
- there are two pathways from here but
we will list only one
- beta decay toThorium-227 (227Th90,
[1.9 days])
- alpha decay to Radium-223 (223Ra88,
[11 days])
- alpha decay to Radon-219 (219Rn86,
[3.9 seconds])
- alpha decay to Polonium-215 (215Po84,
[2 milliseconds]) - two paths again, only one described
- alpha decay to Lead-211 (211Pb82,
[36 minutes)
- beta decay to Bismuth-211 (211Bi83,
[2.2 minutes]) - two paths again, only one described
- alpha decay to Thallium-207 (231Tl81,
[5 minutes])
- beta decay to Lead-207 (207Pb82,
)
- Lead-207 is stable
- some of the decay steps above also
release gamma radiation
- the numbers
in square brackets are half-lives,
the amount of time it takes for half of the atoms of an isotope
to decay
Fission
- Fission
is triggered by a neutron colliding with and splitting the nucleus of a heavy
atom like Uranium-235
- 235U92 is
a rare isotope (the most common type is 238U92)
- The
nucleus splits, releasing three neutrons, daughter atoms (which can be radioactive),
and a large amount of energy.
- The
two smaller nuclei formed are Barium (142Ba56)
and Krypton (91Kr36)
- the energy we can use to heat water and
generate electricity
- [note
- I can't write the atomic number and atomic mass to the left of the symbol
as is usual so I am putting the atomic mass to the right as a superscript
and atomic number to the right as a subscript
- A
nuclear chain reaction occurs
when the emitted neutrons hit other nuclei.
- Since
there are three neutrons emitted when U235 fissions,
three more U235 will
split, emitting nine neutrons, splitting nine U235 and
releasing 27 neutrons, and so on and so on - this is a positive feedback
loop.
- A
chain reaction will not happen if the neutrons hit other nuclei that do not
emit neutrons when hit
- These
other materials may absorb the neutrons without splitting (more
on this later) and so, the density of fissile nuclei controls whether
or not a chain reaction
begins
- the
fission of a nucleus must, on average, produce the fission of more than one
other nucleus for a chain reaction to happen
- Unstable
and stable nuclei still have to “ climb an energy hill" before
the nucleus will fall apart (like an energy of activation for chemical
reaction)
- Nuclear
Fission and Radioactive Decay are not the same thing.
- Nuclear
Fission produces no alpha, gamma, or beta radiation but but does release
high-speed neutrons as radiation (very damaging)
- Radioactive
decay is a spontaneous result of intra-nuclear instability
- Fission
depends on a collision with an external particle
- Neutrons, since they are electrically neutral,
have the penetration power of gamma radiation
Controlling
Fission
- To
induce a sustained nuclear chain reaction, one must increase the probability
that excess neutrons from one fission event will cause other fissionable
atoms to split.
- Moderators
like graphite, beryllium, and water slow the speed of neutrons without absorbing
them.
- A
modern nuclear reactor core contains fuel, a moderator, and control rods
(cadmium or boron) to permit a sustained but controlled chain reaction.
- Light
Water Reactor
- The
water of the primary system, which serves as a moderator and may become radioactive,
is entirely housed in the containment vessel.
- The
secondary water system is heated by the primary water loop and produces the steam
which drives the turbine generator.
Nuclear Fusion
- The
Sun generates heat and light by fusion.
- Fusion
is the result of gravity pulling hydrogen into center of Sun, where
it is squeezed together so tightly, fusion occurs
- Some
nuclear weapons use fusion reactions
- Fusion
in bombs is initiated by conventional explosives surrounding fusible material
the force the hydrogen atoms together
- By
colliding deuterium and tritium, a fusion of the separate nuclei (helium)
occurs, releasing a neutron and energy
- Extremely
high temperatures and pressures are needed to fuse the nuclei of light elements.
- The
first artificial reaction was attained on Earth in 1954—with the detonation
of the first
hydrogen bomb.
- Controlled
and sustained fusion is still in the development stage and not yet economically
feasible as a commercial energy source.
- Fusion
power plants must overcome some problems
- How
to initiate the fusion
- Lasers
have been suggested as a means of initiation (heating and compressing)
- How
to contain and control the fusion
- Magnetic
fields have been suggested as a means of containment
- Some
see fusion as an ideal energy source because:
- We
are not likely to run out of fuel
- Fusion
does not produce fissionable materials that could be used in bombs
- The
high temperatures generated by fusion could be used to vaporize all waste
materials into their component atoms
Advantages and Disadvantages of Nuclear Power
- Extracting
and processing uranium for use in nuclear reactors has several environmental
impacts: mining, tailing production, and contamination of land and water
by toxic metals and radioactive nuclides.
- Uranium
resources, like fossil fuels, are nonrenewable and high-grade ore sources are
being depleted.
- It
is difficult to determine what our nuclear resources are as exploration has
not been extensive.
- Breeder
reactors (not used in the US) can extend the life of fuel by 60 times by “
breeding” more fissile nuclei than the number of nuclei that actually
undergo fission.
- Ratio
of nuclei that fission to those produced can be 1 to 1.8
- U-238
has 92 protons and can capture another neutron (from the fission of U-235)
to become U-239
- U239
undergoes two successive beta decays, and each one converts a neutron into
a proton (and the ejected electron)
- The
first beta decay changes U239 into Np239 (Neptunium)
- The
second converts Np239 into Pu239 (Plutonium)
The
Safety Record of Nuclear Power (from the textbook, amended by me)
- Nuclear
power generation has thus far proven to be the safest form of large-scale
power generation by some measures.
- However, “Black
Swan Events” (catastrophic failures) make nuclear power very risky
- The
book says that "The economic and health impacts of nuclear power are lower
than the impacts from coal-fired power plants."
- this
is so if you consider carbon emissions but it is a very broad statement and
I don't know that I agree
- If Chernobyl has increased cancer rates for
millions and caused many thousands of deaths, it is hard to see nuclear
power as significantly better than any other non-renewable resource
- Nuclear
Plant Accidents
- Five
nuclear cores have undergone total or complete meltdowns
- One
at Three-Mile Island in PA
- one
at Chernobyl in the USSR (now in the Ukraine)
- three
at Fukushima, Japan
- Chernobyl
- Operator
error caused the power plant explosion at Chernobyl, the worst nuclear disaster
so far. (remember that your text was written before Fukushima)
- The
supervisor of the Chernobyl damage control team suggested that perhaps 35
million people were damaged by high levels of radiation following the accident.
- How
many died at Chernobyl?
- 2
died in the explosion
- 237
suffered from Acute Radiation Sickness and 28 died as a result
- 29
died as a result of other causes related to the disaster
- Estimates
for the number of cancer deaths from exposure to the released radioactive
materials range from 9000 to 985,000
- Three
Mile Island
- The
Three Mile Island accident in the U.S. (triggered by a combination of operator
and design errors, a stuck valve, and faulty sensors) produced much less
damage.
- Fukushima
- No
one worried about tsunamis until Fukushima
- The
nuclear power plant there was designed to withstand earthquakes but not tsunamis
- This
lead to two disastrous situations
- The
storm walls protecting the plant from waves were too small
- The
gas-fueled generators needed to supply electricity to run the plant safely
during an emergency were put into the basements
- When
the tsunami came (generated by an off-shore earthquake) the flood walls were
topped and the water ran into the basements so that there was no way to control
the nuclear reactors
- uncontrolled,
they began to heat up and to generate hydrogen gas, which eventually exploded
and worsened the disaster
- eventually,
water was pumped into the reactor cores to moderate them and reduce the heat
output to manageable levels
- those
who restored the control risked their lives to do so
- the
event was close to becoming a melt-down, where such heat is generated that
the nuclear fuel is expelled over a large area and into the air
Drawbacks
of Nuclear Power
- There
are two main technical drawbacks
- 1.
resources need to extract uranium, building plants, and dealing with the
pollution generated
- 2.
safely handling the radioactive waste.
- Enormous
amounts of energy, land, and materials must be used to build the plant.
- More
resources are used to prospect for, mine, and process nuclear fuel
- routine
operation creates thermal pollution.
- Cooling
the hot water produced by the plant can warm nearby rivers and lakes
- After
30 to 40 years, an aging nuclear power plant must be decommissioned, another
energy and resource intensive operation.
- There
is still no long-term, satisfactory method for disposing of the radioactive
wastes generated by nuclear power plants.
- Radiation
- Radioisotopes
or radionuclides are unstable atoms that give off radiation while undergoing
spontaneous disintegration.
- Radiation
will affect any atoms or molecules it encounters.
- Rapidly
growing and dividing cells are usually most affected by radiation.
- There
is no “threshold” of exposure necessary to produce potentially
deleterious results.
Safer Nuclear Reactors
- The
typical nuclear reactor is very large and complex.
- This
complexity makes them expensive, difficult to build, and prone to error
in construction and operation.
- The
larger the reactor, the larger the potential catastrophe if something malfunctions.
- Designs
to minimize these concerns include:
- Smaller
scale
- Passive
safety systems
- Modular
design
Nuclear
Waste Disposal
- Although
massive amounts of radioactive wastes have been generated since the 1950s,
there is still no
satisfactory plan for disposal.
- Most
high-level wastes are currently stored “temporarily” on the reactor
site in pools of water.
- Low-level
wastes are buried in shallow landfills.
- There
has been a geometrically growing accumulation of nuclear waste and steady
growth in the rate of production of waste.
Disposal
Problems
- Many
reactor sites are running out of “
temporary” disposal space.
- Safe
permanent disposal requires:
- Perfect
containment with no leakage
- Guarding
to prevent political or terrorist diversion for potential weapon
use
- Secure
transport to a permanent repository
- Proposals
to dispose of waste include:
- Rocket
loads of waste launched into the sun
- Deep
sea burial
- Burial
in Antarctic glaciers
- Burial
in stable geologic zones
Decommissioning
Nuclear Reactors
- Exposure
to high temperatures and radiation bombardment limits the life
of reactor components.
- The
probable life span of a reactor is between 30 and 40 years.
- Recent
20-year operating license renewals for plants built in the 1960s will postpone
decommissioning but may increase the risk of major
accident.
- The
complete cost of decommissioning a nuclear power plant is still unknown.
Global
Nuclear Power Today and in the Future
- Around
the world, new nuclear reactors are being built while older reactors
are taken off line.
- In
North America and most of Europe, the active construction of new plants
is virtually halted.
- In
Asia, many countries are still actively developing nuclear plant capacity.
- June,
2011 - The Tennessee Valley Authority has agreed to investigate the feasibility
of modular mini-nuclear reactors on the site of the abandoned
Clinch River
Breeder Reactor in Oak Ridge
- Two
of the new Babcock & Wilcox-designed "mPower reactors" by
2020.
- Each
of the new reactors would produce 125 megawatts of electricity --
about 10 percent as much as most conventional reactors -- and could be built
in controlled factory conditions to cut production costs and
ensure construction quality.
- The
mPower reactor technical details:
- The
mPower reactor will use 5% enriched uranium in fuel rod assemblies which
are similar in design to those used in
1,000 MW plants.
- At
a price of $3,000/Kw, a single unit would cost $375 million
- One
of the intended uses of the mPower reactor is to “repower carbon-intensive
plants where the transmission and distribution infrastructure is already in
place. (coal to nuclear ? )
- First
units could be received by customers by 2018 and the reactor is be shipped
by truck and rail
to a site and installed below grade by skilled trades without
complex training.
s22
Last updated April 18, 2013