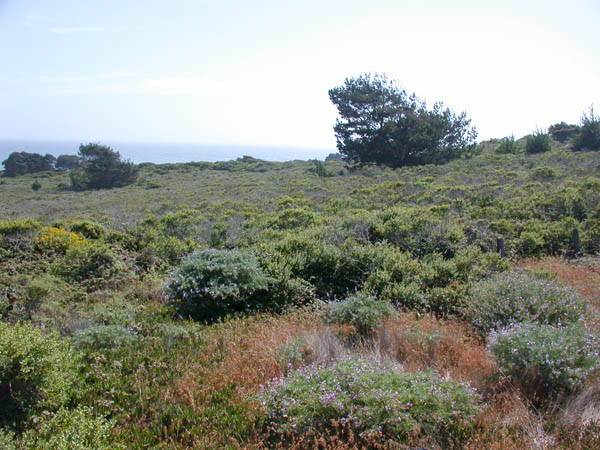
|
BIOL 4140
Contemporary Problems
in Environmental Science
Phil Ganter
302 Harned Hall
963-5782 |
Chaparral overlooking
the Pacific coast |
Energy II: Renewable Energy Sources
Email
me
Unit
Organization:
Reading:
Textbook: Chapter 8
Renewable Energy Sources
Renewable
means that the energy available from a renewable source is not depleted by
using the source
- Hydropower
(including waves, currents, and tides)
- Biofuels
(including both energy crops and burning plant material for heat and light)
- Geothermal
Power
- Solar
- Wind
Four
things to remember about renewable (alternative) energy sources
- There
are always environmental costs but the costs vary greatly among alternative
sources
- Alternative
sources can be either hard or soft technologies and we will discuss both
- All
of
these technologies are underutilized for various reasons
- The
potential for energy production varies greatly among renewable sources
World Renewable Energy Production in 2008 (Wikipedia, but checked with other
sources)
Source |
Production (GW) |
% All Renewables |
% All Energy
|
Large Hydropower |
860 |
51.63 |
9.809 |
Biomass Heating |
250 |
15.01 |
2.851 |
Solar Heating |
145 |
8.70 |
1.654 |
Wind Electricity |
121 |
7.26 |
1.380 |
Small Hydropower |
85 |
5.10 |
0.970 |
Ethanol Fuel |
67 |
4.02 |
0.674 |
Biomass Power |
53 |
3.12 |
0.593 |
Geothermal Heating |
50 |
3.00 |
0.570 |
Solar PV Grid Electricity |
13 |
0.78 |
0.148 |
Biodiesel |
12 |
0.72 |
0.137 |
Geothermal Electricity |
10 |
0.60 |
0.114 |
Solar Steam Electricity |
0.5 |
0.03 |
0.006 |
Ocean Electricity |
0.3 |
0.02 |
0.003 |
Biomass Total |
381 |
22.87 |
4.346 |
Totals |
1665.8 |
100 |
19 |
- Solar PV refers to electricity from photovoltaic panels and Solar Steam refers
to electricity generation by concentrating solar energy to boil water
- Biomass Power refers mostly to electricity from biogenic natural gas but also
includes any liquid fuel production apart from ethanol and biodiesel
- Biomass sources include biomass heating, ethanol,
biomass power, and biodiesel
Renewables are becoming important as a means for economies to develop
- China, India and Brazil, some of the most dynamic and growing economies
have all invested in renewable energy (although the type of energy
production varies among countries)
- US Public support for energy companies has been around for many years
- The current debate is not over should there be support, but which parts
of the industry should get the support and how much
- From the last lecture, we know that renewable energy gets only about 18
% of the US investment
- Fusion,
which has yet to generate enough electricity to power a light bulb, gets
almost as much as all renewables
US Renewable Production
Power Source |
Units in Operation |
Power Capacity (GW) |
% of total Capacity |
Annual Energy (billion kWh) |
% of annual production |
Hydro |
4,171 |
98.7 |
8.7 |
255 |
6.1 |
Wind |
689 |
39.5 |
3.5 |
95 |
2.3 |
Wood |
346 |
7.9 |
0.7 |
37 |
0.9 |
Biomass |
1,574 |
5 |
0.4 |
19 |
0.45 |
Geothermal |
225 |
3.5 |
0.3 |
15 |
0.36 |
Solar |
180 |
0.9 |
0.07 |
1 |
0.02 |
Total |
7,185 |
155.5 |
13.7 |
422 |
10.1 |
- Note that the US has no appreciable contribution
from ocean power, wood refers to wood used to produce electricity, that
solar here includes both solar categories
above, and that biomass in this table is
ethanol,
biogenic natural gas, and biodiesel
- The US produces, as a percentage of its total, about half of the total renewable
energy as the world in general
- much of the difference results from the fact that the US does no heat with
biomass
Footprints:
- New energy sources start with heavy economic disadvantages
and many governments choose to invest in new technology before it is truly
competitive
- Renewable energy gets investment by national governments
- China invests most, US second so investment
is not limited to developed countries
Hydropower
The
book restricts this topic to electricity produced by dams but I will include
all water-based power generation
- Water
powered man’s industry thousands of years ago in the form of
a Water Wheel
- Water
wheels and turbines work on the same principle
- blades
are pushed by moving water and the blades turn the shaft to which they are
attached
- water
wheels used by Romans and Greeks 2000 years ago to grind corn
- by
the 1700’s,
factories were powered by water wheels
- in
1872, hydropower for electricity production began in Appleton, Wisconsin
at 12.5 kilowatts
- that’s
125 100-watt incandescent light bulbs
Hydropower
may come from
- Dams (gravity supplies the force to move the water)
- Tides (gravity of the moon and sun supply the force to move the water)
- Waves (wind supplies the force to move the water)
- Streams or Rivers (gravity
supplies the force to move the water)
- Temperature or Salinity
Gradients (potential
energy in the gradients generate electricity)
Hydropower
from Dams
Dams
are built
for several purposes:;;
- Generating
electricity
- Flood
control
- Water
Storage
- Recreation
In
the US (according to the Army Corps of Engineers)
- There
are over 79,000 dams (and many more too small to make the list
- There
are 2,540 dams that generate electricity
- There may be as many as 5000 sites for new dams
that produce hydropower with the capability of doubling the total electricity
- This could double the electricity generated by dams
- Currently, about 7%
of electricity is hydro, so the potential is only 14% of our electricity
demand TODAY and as demand goes up, hydropower could supply less and
less of that demand
- We will not solve the energy crisis with dams
Dams
can be removed if their costs are greater than their benefits
American
Rivers lists 925 removals over the last 100 years
Tennessee
ranks 7th in hydropower production (WA and OR are top 2)
- The
history of dam building in TN is interesting and is reflected in many current
debates over
the role of the federal government in our society (hint for paper
topic) and involves the Tennessee Valley Authority (TVA)
Update
on the Three Gorges Dam
- on
the Yangtze River in China (largest hydropower project ever)
- Project
is essentially complete
- Will
produce 22,500 megawatts of power (US produces 74,000 megawatts total hydropower)
- Dam
is 1.5 miles wide
- Flooded
400 miles of river
- Displaced
1,300,000 people
Impacts
of the dam
- Running
at full capacity, it
- Reduces
coal consumption by 31 million tonnes per year (tonne means metric
ton, 1,000 kg or about 2,200 lbs)
- Avoids
100 million tonnes of greenhouse gas emissions (CO2)
- Avoids
the release of
- 1,000,000
tonnes of sulfur dioxide
- 370,000
tonnes of nitric oxide
- 10,000
tonnes of carbon monoxide
- a
large amount of mercury
- It
saves the energy needed to mine, wash, and transport the 31 million tonnes
of coal from northern China.
- Agriculture
upstream deposits lots of silt into the Yangtze
- Much
will sediment out in reservoir
- Loss
of silt may cause delta of Yangtze to erode (site of Shanghai)
- Dam
is built on a fault line
Advantages
of Hydropower from Dams:
- efficiency
- low
cost (after initial investment)
- carbon-neutral
(after initial investment)
Disadvantages
of Hydropower from Dams:
- Initial
investment very costly
- Flooding
of land adjacent to the river
- Reservoirs
(the lakes created by dams) fill up due to sedimentation from incoming water
(both river silt and runoff from land next to reservoir)
- Reservoirs
affect water quality
- During
dry periods, downstream flow may be severely reduced
- Interruption
of river migration
- Anadromous fish
grow to adulthood in the ocean but migrate into freshwater to breed
and the young fish return to the ocean (salmon, shad herring, some
sturgeons)
- Catadromous fish grow to adulthood in freshwater and migrate to the ocean to breed
(the young or larval forms migrate back into freshwater) - freshwater eels
are catadromous
- new dams often displace people
- if few people live there, the area flooded is
often a valued natural or scenic area
- limited number of places to put dams
The
future of hydropower from dams:
- smaller
facilities may minimize environmental impacts
- new
technology that allows for electricity generation without dams
that sluice water past turbines
- Diversion or Run –of-the-River facilities
- Topology of the river must
be right
- Small turbines with no dam
- No reservoir needed
- Animal migration impact reduced
- No change to sediment load
- No water storage or flood control
- Adding generating capacity to existing dam
- Upgrading existing plants to
increase their capacity through improved technology
- Worldwide, hydropower from freshwater is likely
to increase over the next several decades but it is unlikely to contribute
as much energy as
the fossil fuels have or to meet increases in demand as more countries
develop.
Alternative Hydropower
Ocean
Energy is the other source of Hydropower
- Commercial
ocean energy developments have been based on both the movement of water
and on differences
in salinity
or temperature.
- Both wave
action and tidal flows contain tremendous amounts of kinetic energy and
are being used to generate electricity.
- Gradients
in salinity (where fresh meets salt water in estuaries) or temperature
(ocean surface versus deeper, 4°C water) can generate electricity
Potential
of Alternative Hydropower
- DOE Reports
assessing the national potential for hydropower conclude that there
is great potential for Wave and Tidal Energy Production near U.S. Coasts
(January 18, 2012)
- waves and
tidal currents off the nation's coasts could contribute significantly to
the United States' total annual electricity production
- provide
clean, renewable energy to coastal cities and communities.
- water power,
including conventional hydropower and wave, tidal, and other water power
resources, can potentially provide 15%
of our nation's electricity by
2030
- The
United States uses about 4,000 teraWatt hours (TW) of electricity
per year.
- DOE
estimates that the maximum theoretical electric generation
that could be produced from waves and tidal currents is
approximately 1,420 TW per year, approximately one-third of the
nation's total annual electricity
usage.
- Note that
not all of the resource potential identified in these assessments can realistically
be developed
- Expansion
from the 6% of the nation's electricity already generated from
renewable hydropower resources is very likely
- Most US coastal areas have potential
to generate electricity from both tides and waves
- Exceptions are the Gulf Coast and the
southern Atlantic coast, where waves are less energetic
Ocean
Thermal Energy Conversion (OTEC)
- Potential
energy available is large but technology is not ready
- There are two ways of generating electricity
from the ocean's temperature gradient
- Closed OTEC systems
- Hot
surface water used to heat colder water
- Cold water is held in a rigid container
- Heating
causes expansion of cold water and puts pressure on container
- Pressure forces heated
water through a turbine, generating electricity
- Open OTEC Systems
- Hot,
surface water used to evaporate (boil) water or another "working
fluid" and create steam
- Steam drives turbine
- Cold,
subsurface water used to condense steam so it can be recycled and
heated again
- Sometimes
the “working fluid” is ammonia or a hydrocarbon
- If water
is used, the condensed water is suitable for drinking or irrigation
- Potential
is largest where the surface water is warmest, which means the tropics
and, especially, the hot areas that determine ENSO
- Can be based
on land, floating offshore or on shallow ocean bottom (continental shelf)
- First
thermal plant was built in Cuba in 1934 (22 kW) but all built since
then have
been for research
Tidal and Wave Power
Tides
are the distortion in shape that result from the gravitational attraction
between objects and they are complex (below is a bit of a tidal primer)
- We
normally use the term to refer to the distortions of the Earth
and Sun' effect on one another and Earth and Moon's effect
- Tidal forces are produced by the (slight) differences in
the Moon's (or Sun's) gravitational effect in different places on the Earth
- The
Moon's effect on the Earth is greater than the Sun's tidal effect
because, even though the Sun is much more massive than the Moon, it
is also much
farther away
- The
force of gravity declines as the square of the distance
and the differences in the gravitational effects that produce
tides actually decline as the
cube of the distance
- Tidal
forces are experienced by all parts of the Earth (inside and outside)
but not equally over all parts of the Earth
- As
compression and expansion forces on solid rock
- Water,
of course, is a non-compressible fluid, so ocean water moves when
the tidal force is applied
- Most
coastlines get two tides a day
- The differences in tidal force produce a
net force in the direction of the Moon on the side of the Earth facing
the moon
- Because the direction of the force changes
as you move closer to the line passing through the center of the Moon
and Earth, the net effect on water produces a bulge with its peak directly
under the Moon
- However, the tidal forces produce a net
force in the opposite direction on the opposite side of the Earth
- This produces a second bulge with its
peak also on the line between the center of the two bodies
- Tidal
bulges remain where the Moon-Earth
axis is, and the rotating Earth slides under the bulges
- The
tidal bulges are at "fixed" points in the Earth-Moon system
but the Earth is also rotating underneath the fixed bulges, so it takes
about 12 hours for a point on the Earth under one bulge to rotate to
be under the other bulge
- The
time between high tides is not exactly 12 hours
- The
Earth-Moon system is rotating (in the same direction as the Earth's
spin rotation) while the Earth rotates
- By
the time the Earth's spin takes a point on its surface from under
one bulge to under the other, the bulge on the other side
has moved due to the Earth-Moon system's rotation
- The motion of the bulge means that it takes
a little over 12 hours for the Earth's rotation to move a point on
its surface from one tidal bulge to the opposite bulge (it takes about
12 hours and a little over 24 minutes
- So, if high tide is at noon today, then
the next high tide is at 24 minutes past midnight and the one
after that is at 12:49 tomorrow
- There is great variation in tidal height and periodicity in different
places
- Imagine the Earth as a smooth ball with
a uniform layer of water covering it
- Under these conditions, the tides would be
very uniform but a bit delayed (the friction between the water and the
bottom of the ocean would create a bit of drag)
- However, there are continents in the way and
they have irregular shapes and the water sloshes against them
- In a smaller basin (like the Caribbean Sea)
the sloshing can set up standing waves that affect tide height and timing
- Complex basin morphology and location on
Earth (tidal forces change direction, remember) can both
- Dampen
and increase tidal height (up to 45 feet in the Bay of Fundy, in Canada
just north of Maine and 40 feet in Rance, France)
- Make one tide a day higher than the other
up to the point that one high tide is canceled out
(some places have only 1 tide a day)
- Interaction
between the tidal forces caused by the Sun and Moon causes variation
in the high tide mark over the course of a lunar month
- Highest
tide is the Spring Tide, when Moon and Sun align
- Lowest
high tide is the Neap Tide, when Moon and Sun are at right angles
Tides
can be used to drive underwater Turbines attached to vanes
- These
images are from a Marine Energy Park being built by the United Kingdom between
Bristol and the Isle of Scilly
- Planned
capacity by 2050 is 27 gigawatt
Tidal
Barrages dam the tides both coming and going
- A
barrage is a way to use the difference in wave height between low and high
tide.
- Essentially
a dam along an estuary
- As
the water level is lower on the ocean side of the barrage, the water wants
to return to equilibrium.
- By
forcing the water to flow past a turbine, energy is generated when the
tide is low and water moves from the “basin” or estuary side
to towards the ocean.
- Opposite
happens when tide is coming in and the Ocean side is higher
- The
use of tides to power water mills (tidal mills) to grind flour or power
a manufacturing process is 2000 years old but the Rance Tidal Barrage in
northern France (Brittany)
is the first to generate electricity
- Completed
in 1966
- 240
mW peak generation capacity
- 8
m to 14 m tides
- the
number of sites which are capable of supporting a Tidal Barrage is limited.
- All
sites are in estuaries where the daily tidal change is large
- Tidal
barrages have many environmental impacts on estuaries (recall that estuaries
are vital areas
for many fisheries)
- Changes
pattern of flow and silt deposition in the estuary
- Changes
salinity and temperature gradient within the estuary
T-shaped
barrages proposed method of using tides along coast
- Most
tides don’t
move perpendicular to the coast but at an angle so that they may be said to
move along the coast
- T-
shaped Barrages that stick out from the coast trap the tide on one side
- As
level rises on one side, water flows through barrage sluices and drives turbines
- Not
yet built but may greatly expand the number of sites where tidal energy can
be harnessed
Sluices
(Gated Channels) that narrow the water flow work without the barrage
- A
sluice allows water to flow along a path that gets ever narrower
- Water
must flow faster and faster and can be made to turn a turbine
- Less
environmental impact than a barrage system
- Can
be placed in tandem so that total energy potential is large
- Can
be used in rivers as well
- 500
kW units are already operating in Humber Estuary in England
Environmental
impacts
- fish
and invertebrates can be killed by turbine
- changes
flow pattern in the estuary or river
Hydro
Energy Barrels (HEBs)
- It’s
a waterwheel driven by tide
- Can
also be used in streams or rivers
- Micro-power
system for individual uses
- Can
also make use of wave action
Wave Power
Waves
are powered by winds and are available 24 hours of the day
- As
wind varies, so do the height and frequency of waves
- Both
affect the power available
- Have
to construct equipment to withstand extreme events
- Lots
of energy but spread out over large area
- Several
different approaches to generating electricity have been devised and most are
being used, even if only
as demonstrations
- Some
are on land, most are offshore
- the
kinetic energy of the wave is used to turn a turbine that spins the shaft of
a generator
Compressed
Air Systems
- Waves
push water under lip of capture chamber
- Extra
water rushing in compresses air which is pushed through the fans of a turbine
- As
water recedes, air pressure is reduced and the air flows through the
turbine in the opposite direction
- Sites for this sort of generation are limited
but environmental impacts are very low
Wave
"Worms" ? Electric Eels?
- Waves
bend these segmented "worms" - long buoyant tubes
- Bending
segments pull and push pistons on adjacent segments
- Pistons
pump up hydraulic pressure and the pressure forces fluid through a turbine
Waves
can fill onshore reservoirs
- Waves
break over a weir and water remains above sea-level
- Reservoirs
are stored (potential) energy that can be released by allowing gravity
to pull the water back to the level of
the ocean
- This
flow can turn a turbine and generate electricity
- The
same idea can fill smaller, offshore reservoirs
Buoys move up and down with waves
- The
vertical motion can be used to pull a coil of wire through a magnetic field
- This
motion will generate electricity
- Lots
of buoys needed to generate significant power but each buoy is inexpensive
and easy to maintain
- Many
buoy designs are in production
- Low
environmental impact
Oyster
Power
- As “shell” of
oyster is moved by waves, water is pumped onto land, where it can be forced
through a turbine or stored in a reservoir
for later
electric generation
- Oysters
are large and hard to position offshore but rather simple devices with few
moving parts
- One
oyster produces 315 kW but the second generation design should produce 2.4
mW
- Little
environmental impact (mostly the permanent disruption of the sea floor)
- Underwater “noise” may
impede communication between individual fish or marine mammals
Osmosis
Power
- Salinity
Gradient can power by osmosis
- Remember, osmosis is the flow of water across
a membrane from where the water is in higher concentration (and solutes
are in lower concentration) to the side with lower water concentration
(and, therefore, higher solute concentration
- Osmosis is powered by diffusion and proceeds
in the same direction (from high concentration to low concentration)
- Osmotic
pressure (as water crosses from fresh water side of a membrane to the salty
side) can be used to build pressure to turn a turbine
and generate electricity
- In
late 2009, a 1 megawatt pilot plant opened in Norway
- Some
of the electricity generated is used to pump the water into the plant
- The
surface of the membranes in the plant are measured in square kilometers
Biomass
- Biomass
provides as much as 15% of all energy consumed worldwide, although only about
3% of energy demands in the US
- In
some developing countries, biomass provides 90% of the energy consumed.
- Heat
generated by the direct combustion of wood or organic wastes is the most
common use of biomass energy.
- Biomass is also used to generate electricity
- Biomass-derived
fuels, like ethanol and biodiesel, are another way this resource is used.
Major
sources of biomass energy include:
- Wastes
(construction, home, commercial)
- Sewage (both biogenic natural gas and combusted
sewage solids)
- Wastes
(industrial) – animal fats, vegetable oil, paper mill waste, various
other sources
- Standing
forests and tree plantations
- Energy
crops (beet or cane sugar, corn sugar and oil, soybean or rapeseed oil, palm
oil)
Biomass
can be used in several ways:
- Direct
combustion
- Thermochemical
conversion to methanol or syngas
- Biochemical
conversion to ethanol or biogas
Concerns about the use of biomass to generate electricity include:
- It is not
renewable if it is harvested faster than it grows
- Some biomass conversion
processes are very inefficient
- Biomass combustion can be a major contributor
to air pollution
Ethanol from corn as an example of biomass energy
and can serve as an example of the how complex the issue of bioenergy may
be
- Highly federally subsidized
industry, so ethanol gas mixtures are cheaper at the pump only because of government
support (say thanks to those who don't drive when filling up!)
- Touted as lowering carbon emissions
- Carbon in ethanol from corn was taken from
the atmosphere, so when the ethanol is burned, it replaces the carbon taken,
making it carbon neutral
- Every gallon of corn ethanol burned is less gasoline
burned
- Impact on carbon emissions less than expected
- Carbon used to produce corn (making
pesticides and fertilizers, transporting corn)
- Conversion of forest to agriculture
and pasture releases carbon to atmosphere
- Impact on those depending on corn
as food
- 20% of corn crop in US is used to make biofuel (ethanol)
- Corn prices have
doubled world-wide
Solar Power
- Much
of the energy we use at present is indirect solar energy.
- All
fossil fuel’s
energy comes from solar energy
- While
people currently consume about 13 terawatts of energy per year, the sun delivers
about 80,000 terawatts to the Earth per
yr.
- 1⁄4
of total is usable
- Direct
solar energy is dilute, diffuse, and intermittent.
- The
large amount of solar energy available could potentially solve many of our
current energy problems.
- Solar's
23,000 tW (teraWatts)/year of energy is greater than all of the energy in the
known coal , oil, natural gas, and uranium reserves total
- However,
that energy is never very dense anywhere, so it must be harvested from a very
large surface area
- Solar
energy is not limited by available energy but by our ability to harvest the
energy to do work for us.
Solar
energy is not uniformly distributed
- Both
latitude and climate effect the amount of energy that gets to the ground
- In
the US, the southwest is the place
What
is the current state of solar hard tech? - here are the largest power plants
as of 2009
- The
average capacity
of coal-fired plants in 2009 was about 236 MW
- Largest
Solar Thermal Power Plants in Operation
- “Solar Energy Systems” in the Mojave Desert CA, USA — 354
MW
- “Solnova Solar Power Station” in Seville, Spain — 150
MW
- Largest
Solar Photovoltaic (PV) Power Plants in the World
- “Sarnia Photovoltaic Power Plant” in Canada — 97
MW
- “Montalto di Castro Photovoltaic Power Station” in Italy — 84.2
MW
- “Finsterwalde Solar Park” in Germany — 80.7
MW
- “Ohotnikovo Solar Park” in Ukraine — 80
MW
Note
the the PV systems are found in colder regions – PV gets more efficient
at lower temperatures
As
of 2012, some regions have begun to seriously exploit their solar resources
for hard tech power production
- Germany
is the leader (54% of total power production in a country not famous for
its sunshine)
- Italy
and Spain are becoming committed (40% and 15%)
- The
US is undercommitted (1%)
- Some
states are committing (NJ and CA at 5% and AZ at 6%)
Challenges
for Solar Power
- Intermittency
is important (cloudy days, nighttime)
- Must
be able to supply energy continuously
- Storage
for night time
- Other
energy sources may be needed
- Distribution
and transmission due to disparity of insolation
- Southwest
gets more sun, but population density is higher in northern US
- Price
- Cost
is mostly for manufacture and installation and loss due to longer transmission
- PV
panels do have finite useful life times but last over 20 years, so costs are
spread out over a long time
Ways
of using
Solar Energy
- Passive
Solar Energy is using the Sun to heat houses, offices and stores.
- Passive
means that there are no special systems or moving parts, the design of the
building
makes the energy
available (very soft technology)
- Active
Solar includes:
- Soft
Tech
- Solar
Panels that produce hot fluids for building heating.
- Photovoltaic panels that generate electricity for local consumption.
- Hard
Tech (large Solar grid electricity generation)
- Photovoltaic
(PV) farms with thousands of panels in a single place
- Solar
Concentration Plants where hundreds of mirrors concentrate sunlight
and heat a fluid to boiling
Passive
Solar
- Passive
solar design employs:
- Solar
orientation
- Thermal
mass to moderate energy release
- Effective
insulation
- Passive
Solar Energy is using the Sun to heat houses, offices and stores.
- Passive
Solar depends on design for a particular climate
- Orientation
of the building and placement of windows
- Natural
movement of air through building
- Takes
advantage of the changing angle of the Sun as seasons change
- Overhanging
eaves
shade in summer but not in winter
- Sunlight
enters through glass and is absorbed by interior of building
- Glass
prevents loss of re-radiated energy (as infrared radiation) through greenhouse
effect
- Energy
is stored in a “thermal mass” to slowly be released during
the night
- Mass may be concrete, concrete block, stone,
or a tank full of water
Active
Solar
- Modern
active solar technology encompasses two themes:
- Trapping
solar heat and light for useful purposes
- Direct
or indirect conversion to electricity
- Sunlight
can be either direct or diffuse.
- Some
technologies require direct solar energy (Thermal) while others (PV) can use
either.
- Active
solar collectors can be used for space heating in buildings where passive
design is not practical or was not used (retrofits)
- Active
Solar includes:
- Solar
Panels that produce hot fluids for building heating.or just hot water for home
use
- This
is not a passive system as the fluid in panels is circulated
- Panels
heat fluid, which can be used to heat water or entire building
- Fluid
might be air or water
- Not
a common usage in the US but, worldwide, a common technology
- China
leads the way, with 2/3 of the installed capacity and Europe has another 1/8
Solar
Thermal
- Environments
with large amounts of direct solar radiation can also use focusing collectors
distributed in large arrays or as power towers.
- The
high temperatures produced by these collectors can be used for process
heat or to generate electricity.
- By
concentrating sunlight, water or another material can be heated and the increase
in pressure used to turn a turbine and generate electricity
Photovoltaic
Panels
- Photovoltaics
use semiconductor technology to generate electricity for direct use or to charge
hydrogen fuel cells.
- Photovoltaic
panels can be soft tech (generate electricity for local consumption) or
- Hard
Tech (large Solar grid electricity generation)
- PV
farms
How
Photovoltaic Cells work
The
Band Gap and Semiconductors
- When
electrons in a substance absorb a photon, they may have enough energy
to leave the nucleus they are moving around
- Valence
electrons (the outer electrons) have low energies and are trapped by
the nucleus but, because they are the outer electrons, they have higher
energies than other electrons in that atom
- The energies of the valence electrons span the
Valence Band of energies
- Higher
energy electrons can move from atom to atom (those electrons are in the
Conducting Band)
- The
valence bands and conducting bands vary in different substances
- Metals
(like copper) may have valence bands that overlap with conducting bands (that’s
why they lose electrons so easily and make good conductors)
- Other
substances may have a gap between the V and C bands, referred to as the Band
Gap
- The
larger the band gap, the less likely an electron will get enough energy
to leave, and the better insulator the material will be
- Semiconductors have
narrow band gaps that can often be jumped by the absorption of
a light photon
Silicon
Semiconductors and Doping
- Pure,
crystalline silicon is a semiconductor
- It’s
band gap will be affected by the impurities in the crystal, so that different
formulations
will
require more or less energy to jump the band gap
- Thus,
layers of differently doped silicon will absorb different parts of the visible
spectrum
- This
means the cell will use more of the available energy
- Photovoltaic
cells are semi-conductors, like some of the components of your computer
- Semi-conductors
are neither good conductors nor insulators, but in-between
- Silicon
is a semiconductor that can be grown as a very uniform crystal
If some improprieties (other elements) are included in the crystal (Doping the crystal), useful electric properties are obtained
- Depending
on the type of impurity, either unpaired, easy to free electrons or positions
in atoms that can be filled by electrons
(called “holes”)
result
P-N
Junctions allow a current to flow
- Some
doped silicon has a natural excess of free electrons (n-type,
for negative)
- Other
doped silicon will have places (called holes) where electrons can
fit into atoms
(p-type, for positive)
- When
two semiconductors of opposite types are layered next to one another,
electrons can flow from the n-type to fill holes in the
p-type, producing a
layer between the two types
- This
is a layer of
neutral charge and is called the depletion region because the excess charge
is depleted in the region
- When
a voltage is applied to opposite ends of the crystal, two possibilities
arise with different outcomes
- If
the n-type is the positive side of the circuit, electrons will
be drawn to the far edge of the crystal and holes will move to the
opposite,
nEGATIVE side of the electric circuit, increasing
the size of the depletion region
- In
this case, the circuit can’t be completed
and no current flows
- If
the n-type gets the negative side, its electrons are pushed toward
the depletion zone (and holes toward the zone as well)
- Now
electrons cross the depletion zone and current flows
- So, the P-N junction acts as a one-way gate,
allowing current to pass in only one direction
Photovoltaic Effect
- With
this background we are now ready to understand how photovoltaic cells generate
electricity
- When
photons are absorbed by electrons in the depletion zone, they are freed to
move (and have the energy to do so) and the leave a hole behind
- The
one-way nature of the PN junction means the electrons can only
leave the silicon in
one direction, which causes a one-way flow of electrons (thus
an electric current is created)
- The opposite effect (applying a charge across
the junction) results in a photon being freed (which means the semiconductor
emits light - the basis of the LED
Environmental
Impact of Solar Energy Production
Positive
- Sunlight
is a fairly perfect abundant, renewable energy resource
- Post
construction, no air or water pollution is produced
- Dispersed
nature of resource can be matched by dispersed generation of power
Negative
- Large
tracts of solar power plants can be disruptive to ecosystems like the desert
biota.
- 1
km2 per 20-60 mW (Union of Concerned Scientists)
- Production
and deployment of solar cell arrays use fossil fuels and other poisonous
chemicals.
- Doping
of silicon can involve arsenic, cadmium, and other harmful metals and
involve toxic organic solvents
Future
of Solar Energy
- Costs
are dropping and technology is improving, so that solar panels may be a much
more important source of electricity than they are today
Wind Power
Origins
- Wind
power is also sun power, as the sun powers the wind
- Wind
turbines were invented in1891 and generated commercial electricity by
1887
Current
Status
- In
2005 (when the textbook came out), wind power generates less than 1.5-2% of
the world’s electricity and is now between 2.5 and 3%
- Its
use is growing rapidly and has averaged 25% per year.
- The
cost of wind-derived electricity has dropped dramatically in the last decade.
- Land
Based Wind farms now generate electricity at competitive prices.
- In
the U.S., as much as 20% of electricity demand could be met by wind power.
- This
figure is not the theoretical maximum, as there is enough wind energy to
power all of the US or the world, but practical considerations limit the
estimates.
- Transmission
capability, storage capability and capacity (next slide) are all
limiting factors
- Improving
technology has increased both the theoretical maximum and the practical expectation
- By 2012, wind power's potential is starting
to make an impact
- Denmark (close to Germany - see solar) - 30%
- Portugal, Spain, Ireland, and Germany - 11%
to 17%
- US still underexploiting at 3% but
- IA and SD near 25%
- ND and MN at 15%
- KS, IA, OK, and OR at 11%
Capacity:
- The
potential for power production from any source will never reach 100% of its
capacity
- Capacity
factors are the % of total possible power that is actually produced
- Each
source has different variables that affect capacity
- Capacity
for wind is reduced by Intermittency and Variability
- Intermittency refers
to those times when insufficient wind to turn the turbine
- Variability refers to the fact that, when the wind is sufficient to turn the blades,
it does not always blow at the optimum speed to maximize power output
Source |
Capacity |
Geothermal |
92 |
Nuclear |
90 |
Gas (Advanced) |
87 |
Coal |
85 |
Biomass Fuel |
83 |
Dam Hydropower |
52 |
Wind |
35 |
Solar (PV) |
25 |
Solar (Thermal) |
18 |
Sources
of Wind and Wind Power
- Most
wind power is over the ocean (specifically, the northern and southern westerlies
- This
favors countries with coastlines, especially at the appropriate latitudes
- North
America, North Africa, Europe, northern Asia, eastern Asia, all have potential
for wind power from their interior regions
- South
America, Sub-Saharan Africa, Australia (note they are at the same latitude)
all have less potential
for wind power from their interiors
- World-wide
generation of wind power has grown exponentially (we know this can only happen
for a limited time!)
- In
14 years, capacity has grown over 30x
- As
developing countries invest, actual capacity has exceeded predictions
- In
the US, potential for wind power generation is not uniformly distributed
- Wind
power potential must consider not just the presence of wind but
its constancy and both the cost and remoteness of the land needed
- The
Midwest has the greatest land-based potential
- Map
is too coarse-scaled to show good local potential but all states have
areas suitable for wind power
- Offshore
production potential is high due to constancy of winds
- Cost
of generation is greater offshore due to higher construction, maintenance,
and transmission
costs
- Transmission is an extra cost when the source
is far from the consumer and many wind power sites are far from population
centers
Price
of Wind Power
- Price
of land-based wind-generated electricity has fallen to near-parity with
fossil fuels in some areas
- Offshore
generation costs are still above fossil-fuel generated costs
- Remember
that these costs do not reflect any of the environmental or health
costs
- Remember
that these costs are somewhat distorted by unequal subsidies for competing
technologies
- Wind
power has become competitive even in though fossil fuels have been more heavily
subsidized by government (local, state and federal)
- The
many factors that affect power prices have lead to many, very different price
estimates
- Some
include potential capacity, some do not
- Some
consider subsidies, some do not
- Almost
none of the price estimates include health and environment
- At
one time, the US lead the world in wind power but that has changed
- China
has become the #1 installer of wind turbines and is now the largest wind energy
producer
- China
installs half of the new turbines each year world-wide
- India
has also become a major installer
Variables
affecting Wind Power (from Genstan Total Alternative Power)
- The
power generated by wind turbines depends on wind speed but a maximum power
limits the utility of high speed winds
- Actual
power curves in wind tunnel tests closely match theoretical predictions
Wind
Speed
- Theoretically,
faster wind carries more power
- In
fact, limitations of the mechanism mean that there is an optimal wind speed
that maximizes power generation
Rotor
Size
- Larger
rotors, more power
- Expense
goes up with power
- Large
rotors can be in excess of 60m (120 m diameter) and generate 7.5 mW (with
10 mW turbines proposed
Tower
Height
- Winds
are faster the higher you go
- The
higher you go, the more people can see the tower, which means that it impacts
more people
Intermittency
and Variation
- Power
demand and power generation do not always occur at the same time
- The
grid allows redistribution of power, which mitigates the effect of both
problems but does not solve them
- Power
from other sources may be necessary if demand can't be satisfied
- Power
storage is another mitigation strategy
- Small
scale storage
- Large
scale storage
- Fill
reservoirs with water pumped by excess wind power production when production
exceeds demand
- Then generate electricity using hydropower
(very efficient means of generation)
- Use
excess electricity to generate hydrogen for fuel cells and run the
cells when wind power can't meet demand
Noise
- Wind
turbines make noise but not much in comparison to everyday sounds
- However,
turbine noise is constant when the wind is blowing
Designs (from Wikipedia)
- Three
basic designs
- Vertical
axis with large panels to catch wind (Savonius)
- Vertical
axis with blades
- Horizontal
axis with blades (Giromill/Darrieus)
- HAWT (Horizontal axis Wind Turbine)
- Must
be pointed into wind
- All
word done turns shaft in the same direction (not true of VAWT) so these are
the most efficient designs
- Largest
is 135 m (413 ft) tall with rotor diameter of 126 m and generates 7.8 mW
- Best
design when winds come from one direction most of the time
- During
high winds, feathering and brakes stop blades from rotating
- VAWT (Vertical-axis
Wind Turbine)
- Don’t
have to be pointed into wind
- Respond
instantly to changes in wind direction
- As
each blade rotates, part of the rotation is into the wind, so some power is
lost to this design feature
- Largest
has 110 m blades and generates 3.8 mW max.
- Vertical
axis designs rotate more slowly than HWAT at a given wind speed
- This
means that the force of the wind is greater on the mechanism, which increases
stress
and wear
- Innovation
continues
- Students
designed a traffic sign that generates power
- Floating
offshore towers are much cheaper than those sitting on the ocean floor
Environmental
Concerns
- Positives
- Fossil
fuel footprint is small
- Manufacturing carbon production is small
compared to fossil fuel plants
- Auxiliary generators that kick in when
wind is not sufficient increase footprint
- Construction cost is small
compared to fossil fuel plants
- Reliable
technology
- Negatives
- Intermittency
- Capacity
for production will not meet total US demand
- Unsightliness
and noise
- Danger
to birds
- Wind
farms take up lots of land because there must be lots of space between rows
of towers
- Turbulence
behind tower greatly reduces power of next turbine
- Land-use
cost is mitigated if the land between towers can be used
- Orchard
land, fields or pasture lands can extend to tower base
- Soft
Tech Applications
- Much
of wind power is generated by hard-tech wind farms
- There
are many VAWT and HAWT systems designed for small scale, local production
Geothermal Power
- The
energy from Earth’s hot interior can be used to heat buildings directly
or to generate electricity.
- Geothermal
Deposits are regions close to the surface that are hot enough to exploit
for power generation or to use the heat as heat
Four basic types of geothermal deposit
- Hydrothermal
reservoirs
- Areas
where surface water percolates down to hot regions through cracks in rock
- Hot
water is turned into steam, which is used to drive turbines and generate
electricity
- Can
be tapped through closed-loop or open-loop systems (different environmental
impacts)
- Geopressured
brines
- Salt
water with methane dissolved in it (so much that it is considered a gas source)
- Heated
over boiling due to pressure (300 – 400 °F)
- 10,000
to 20,000 feet down (thus the pressure)
- Can
be used to heat water (geothermal) or the hydraulic pressure can be used
directly
- Found
off of the TX and LA coasts
- Hot
Rock and Magma
- No
small or large scale plants use these heat reservoirs as of yet
- Up
until now, all large scale energy production has used permeable rock that
already has water in it
- Here,
the energy is tapped by pumping cold water down a well into close
proximity to the heat source
- Water
must fracture rock so that it can flow to the second well hole and move
back to the surface (as hot, not cold water)
Geography
of the Resource
- As
expected, geothermal sites correlate with edges of tectonic plates and hot
spots
- In
the US, most of the currently sites are in the west
Geothermal
Heating and Cooling
- Soft-Tech Geothermal
- As
you go underground, the temperature increases, but from a starting point that
is below room temperature
- Single
building systems pump either air or water down to the appropriate level and
return it at the desired temperature
- Can
heat home and provide hot water
- Can also air-condition house during hot weather
Geothermal
Environmental Impacts
Positive
- Not
air polluting (except for construction)
- Constant
energy source
Negative
- Potential
is not enough to supply total energy demand
- May
require large quantities of water
- Possible ground subsidence
- Water
may dissolve hazardous substances and bring them to the surface
- Steam
venting may release H2S,
NH3,
CH4 and
CO2l
- A
Binary system is a closed-loop system, which separates the fluids that absorb
the heat from those that generate the electricity
Energy Storage
- A
major obstacle to the widespread use of alternative energy is the problem
of energy storage and transportation.
- Most
electricity is used as soon as it is generated
- Intermittency and Variation may mean that
storage is necessary on a large scale
- Fossil Fuels are naturally stored
- Batteries are a possible option, but large-scale
storage has not been attempted
- Batteries are often bulky compared to the
amount of energy they store.
- Batteries produce hazardous waste and chemicals.
- Small-scale battery storage is useful for "smoothing"
electricity generation for the grid
- Car batteries are an option if electric cars
become common
- Alternatives to Batteries
- Hydroelectric Pumping
- Pump water during periods of excess
generation, use hydrostatic head to turn a turbine and generate
electricity when demand
is large
- 22 gigawatts of storage already exist, built
to store excess energy from slow-to-adjust nuclear plants
- New facilities are expensive and difficult
to get environmental approval for
- Thermal Storage
- Store energy as heat by heating fluids
and use heat to generate electricity
- Short term storage, mainly envisioned as means
for storing excess solar energy
- Compressed Air
- Excess energy used to pump air into underground
caverns, often deep, where the air is naturally heated and more energy
is added
- Hot air turns turbines when released
- Well tested in Europe and, close by, Alabama
- Fuel
cells are
similar to batteries but use hydrogen gas to store energy.
- Use excess energy to generate H2
- the energy is stored in the H2 produced
(usually from splitting water)
- When you need to use the stored energy,
stored H2 is
used by Fuel Cells to generate electricity directly, not using
combustion
- Fuel Cells produce electrons on one
side and absorb the on the other, so that an electric current
is established in wires connecting the two sides
- Splitting H2 to
produce H+ ions
produces electrons
- Combining the H+ ions with O2 and
the electrons produced by the splitting form water, the "waste"
from fuel cells
- Fuel cells may be used for tasks like
powering electric vehicles.
Energy Conservation
- The
simplest and cheapest way of stretching our energy resources and mitigating
energy-related problems is through energy conservation and energy
efficiency.
- Energy
intensity is the ratio of energy consumption to economic output
- A
useful index of overall efficiency
- Can
be lowered while maintaining strong economic growth and high standards
of living
- Many developed economies have become more intense
and are getting more for each watt used
Saving
Energy
Energy
can be saved by:
- Not
converting it from one form to another unnecessarily
- Increasing
the efficiency of energy conversions
- Not
transporting energy unnecessarily
- Increasing
the efficiency with which energy is transported
- Encouraging
conservation
- Requiring
new construction and manufacturing to be efficient
Encouraging
Energy Savings
- Voluntary
versus Mandatory Measures
- Governments
can mandate and/or encourage energy efficiencies.
- Voluntary
measures can be encouraged through education, advertising, and with positive
examples in the popular culture.
- Industry
can also promote efficiency; for example, utility companies promoting energy
efficiency to consumers,
and adopting demand-size management strategies.
- Government
can also encourage efficiency through tax breaks and subsidies.
s22
Last updated April 16, 2013